![]() | ||
Satellite system upgrades will enhance security and resiliency on Gray Eagle UAS upgrade program |
Hughes contract from GA-ASI to for next-generation UAS SATCOM – SatNews
Summary
The Hughes Network Systems recently won a production contract from General Atomics Aeronautical Systems (GA-ASI) to provide advanced, ruggedized HM400T modems for the next-generation SATCOM system on the MQ-1C Gray Eagle 25M Unmanned Aircraft System (UAS).
Key points:
- - The HM400T modems integrate the Department of Defense's Enhanced Bandwidth Efficient Modulation (EBEM) waveform technology and transmission security (TRANSEC) features.
- - This enables increased endurance and resilience for the Gray Eagle 25M's Airborne Intelligence, Surveillance and Reconnaissance (AISR) missions, especially in contested environments.
- - The modems meet low size, weight and power (SWAP) requirements and support government approved waveforms for resilient ISR transmissions.
- - They will help the Gray Eagle 25M UAS support the U.S. Army's demands for future unmanned and manned teaming operations.
- - The HM400 modem technology leverages an open architecture and can operate over multiple frequencies and orbits. It uses Hughes' unique Scrambled Code Multiple Access (SCMA) waveform.
- - SCMA enables bandwidth efficiency for smaller antennas and works well for communications with extremely small micro satellite terminals. It provides advantages over traditional CDMA and TDMA approaches.
In summary, the advanced HM400T modems utilizing the SCMA waveform will provide key communications capabilities for the U.S. Army's next-generation Gray Eagle 25M UAS to enable more resilient and enduring ISR missions.
Hughes Network Systems, an EchoStar (Nasdaq: SATS) company, was recently awarded a production contract from General Atomics Aeronautical Systems, Inc. (GA-ASI) to produce a key component of the next-generation SATCOM)system for the MQ-1C Gray Eagle 25M Unmanned Aircraft System (UAS).
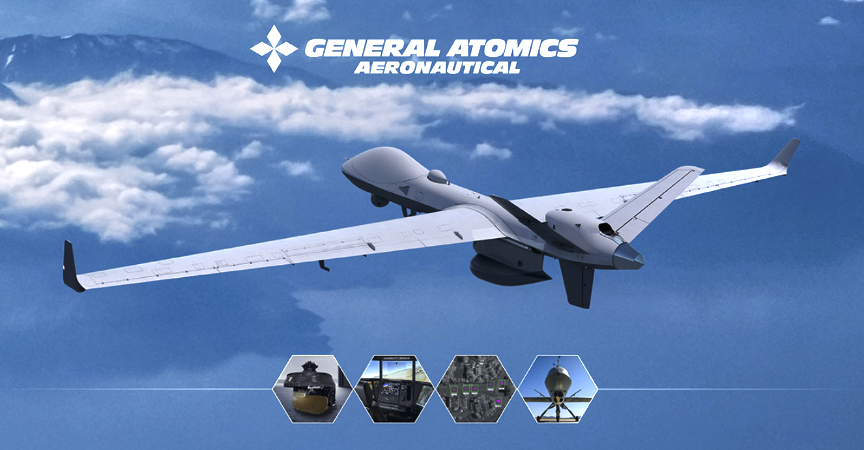
Under the contract, Hughes will provide advanced, ruggedized HM400 (PDF) modems, named the HM400T, that integrate with the DoD’s standard waveform technology and transmission security (TRANSEC) features to enable significantly increased endurance and resilience for Gray Eagle 25M’s wide range of Airborne Intelligence, Surveillance and Reconnaissance (AISR) mission requirements, particularly for operations in contested environments.
Hughes is producing advanced, ruggedized HM400T modems, that integrate with the DoD’s standard Enhanced Bandwidth Efficient Modulation (EBEM) waveform technology and TRANSEC features. This advanced, customized software-defined commercial technology is based on the HM400 modem that is operating on the GA-ASI MQ-9B UAS.
The HM400T incorporates a ruggedized container for high altitude applications, meets low size, weight and power (SWAP) requirements, and supports government approved waveforms to ensure resilient ISR transmissions for the U.S. Army’s next-generation Gray Eagle 25M, MQ-1C, UAS and its long-endurance missions. This next-generation UAS will support the U.S. Army and the U.S. Army National Guard to meet the demands of future unmanned and manned teaming operations and help fill the growing Reconaissance, Surveillance, Targeting and Attack (RSTA) gap. After completing the development phase, GA-ASI awarded Hughes the initial production units; Hughes will start delivering these communications systems in the Spring. This highly advanced UAS platform supports worldwide ISR missions.
“Hughes looks forward to supporting GA-ASI with a secure and cost-effective communications architecture that delivers the speed, flexibility and resiliency necessary to meet the next generation of mission requirements,” said Rick Lober, vice president and general manager of the Defense and Government Systems Division at Hughes. “This production contract reinforces the commitment of Hughes to serving GA-ASI and, by extension, the U.S. Army and this program of record, ensuring enhanced communications and ISR capabilities for today’s multi-domain operations.”
Connect to your warfighters anytime and anywhere with multi-orbit HM System satellite ground technology that’s flexible, scalable and secure.
For the modern warfighter, connectivity is more than a communications line. It’s a lifeline when the certainty of secure communications means everything. It’s also why we invented the Scramble Code Multiple Access (SCMA) waveform to protect SATCOM transmissions in every theater – on a helicopter, aboard an RPA, on the water and on the ground.
Built into our HM System, the SCMA waveform sets apart our software-defined satellite ground capability for military applications. It’s enabled industry firsts, like through-the-rotor, multi-megabit data transmission; multi-orbit SATCOM to and from RPAs; and on-demand, satellite-enabled cellular connectivity for emergency comms.
Flexible and cost-effective, the commercially based HM System meets mission requirements for Department of Defense (DoD) customers with features such as:
- Open standards architecture
- Multiple frequency bands (L-, Ku-, Ka-, Mil Ka, and X-band) and orbits (GEO, NGSO)
- Portable modems with low Size Weight and Power (SWaP)
- Ruggedized equipment able to withstand high altitudes, lightning strikes and Electromagnetic Interference (EMI)
Manufactured in the Hughes factory in Maryland, U.S.A., HM System equipment includes:
- HM400 Modem designed for SATCOM on-the-move with flexibility and resiliency in mind. Ideal for a range of applications, including disaster response, storm tracking, military operations, as well as agriculture surveillance and airborne geological surveys.
- HM100 Hub available for use as a standalone SCPC modem or integrated into a robust, scalable HM Gateway for Communications-on-the-Move capabilities.
HM400 - Hughes Network Systems
The HM400 from Hughes is a Software-Defined Modem that has been designed for satellite communications (SATCOM) on the move. It leverages an open architecture and can be used with any qualified system components that are suited for delivering mission-specific requirements. This modem can operate over multiple frequencies (L-, Ku-, Ka-, Mil Ka-, and X-band) and multi-orbit (LEO, MEO, GEO) constellations. It provides the capability to access worldwide SATCOM services through the existing VSAT and MobileSat service providers or customer-specific networks/gateways. The modem utilizes Hughes’ unique Scrambled Code Multiple Access (SCMA) waveform technology that enables bandwidth efficiency for smaller antennas and EBEM with integrated TRANSEC for fixed and mobile military applications.
The HM400 can host a large suite of downloadable, standardized government, and commercial waveforms. It offers multiple sophisticated signal processing capabilities in a low Size, Weight, and Power (SWaP) footprint. It integrates natural convection cooling mechanisms for temperature regulation and is compliant with environmental standard requirements. This modem delivers a data rate of up to 45 Mbps and an output power of 65-75 W. It has built-in lightning, EMI, and EMC protection circuitry to prevent hazardous events.
The HM400 is available in a ½-ATR-style enclosure that measures 5 x 13 x 8 inches and is compliant with the MIL-STD-810, 461-, 462-, and 464 environmental standards. It is ideal for storm tracking, disaster response, data communications, airborne geological surveys, and Beyond Line of Sight (BLOS) video applications.
Scrambled Code Multiple Access waveform for micro satellite terminals,
L. -N. Lee, M. Eroz, L. Chen and R. Gopal, "Scrambled Code Multiple Access waveform for micro satellite terminals," MILCOM 2012 - 2012 IEEE Military Communications Conference, Orlando, FL, USA, 2012, pp. 1-6, doi: 10.1109/MILCOM.2012.6415683.
Authors
Abstract: This paper introduces a novel waveform based on very low-rate coding and a family of new multiple access techniques named Scrambled Code Multiple Access. It allows different users of a communications system to efficiently share the same bandwidth by using specific scramblers to separate timeslots.
The SCMA technology is especially well-suited for satellite communications with extremely small antenna micro satellite terminals for potential applications, such as, tactical communications on the move, asset tracking, sensor networking, and smart grid. With SCMA, such applications can now be supported with cost-efficient Ku- and Ka-bands.
We will discuss two types of receiver structure, several ways of using them, their respective performance, covertness features, and results from a prototype implementation that has been field tested.
keywords: {Receivers;Satellites;Bandwidth;Phase shift keying;Parity check codes;Encoding;Scrambled code multiple access;interleave division multiple access;low density parity check codes;layered belief decoding;turbo codes},
URL: https://ieeexplore.ieee.org/stamp/stamp.jsp?tp=&arnumber=6415683&isnumber=6415559
Introduction
Satellite-based communication has experienced impressive demand and growth over the last couple of decades in both the commercial, governmental, and military environments. Core satellite technology has made dramatic improvements with innovative and improved coding and modulation techniques, spectral efficiency, Communications on the Move (COTM) capabilities, and increasingly smaller terminal and antenna packaging. Much of the demand can be attributed to the inherent benefits of satellite coverage, where a communications network can cover large geographic areas with a single geosynchronous satellite or a fleet of lower earth-orbiting satellites. These benefits continue to make satellite-based communications an attractive selection for large-scale communication infrastructures.
However, there are challenges associated with the rapid advancement of satellite technology, specifically with respect to mobile and other applications that require the use of Micro Satellite Terminals (MICROSAT) in the Ku and Ka bands. Traditionally, such extremely small terminals have only been available on the L-band (such as BGAN that typically provides about 256 kbps uplink), which has significantly higher operational costs. To enable transmission in and out of these MICROSATs involves a delicate balance of space-segment resource usage, size, and cost of the terminal antenna while complying with emission regulation. This is further complicated by the general desire to reduce the size of the antenna down to previously unthinkable sizes at data rates supporting satisfactory user experiences. All of these challenges are exacerbated when considering the need for scalability from a small pool initially to tens or even hundreds of thousands or above in a large network.
This paper introduces Scrambled Code Multiple Access (SCMA), a unique multiple access scheme that employs new coding and modulation techniques to help obviate the above challenges. In this paper, we start with an overview of the waveform, including comparison with CDMA and Interleave Division Multiple Access (IDMA), and consider the possible role of SCMA in the forward and return link in the context of MICROSAT and include bandwidth and power comparison with DVB-S2. In particular, we describe how an adaptive bandwidth efficiency scheme can be employed using SCMA. Moreover, we demonstrate the superior performance of SCMA as a random multiple access technique. We then highlight some other unique characteristics about SCMA, especially for short burst-sized transmissions, and compare it with CDMA and TDMA. This is followed by a description of a recent MICROSAT implementation that has been field tested for COTM applications. The paper concludes with a thoughtful discussion on the applicability of SCMA technology, in both Industry and Governmental/Military environments.
Overview of SCMA Technology and Waveform
Figure 1 depicts a simple block diagram of an FEC coded SCMA. Suppose there are
SCMA is a variation of (IDMA) where different users that share the same bandwidth and time slots are separated by user specific interleavers [1], [2]. IDMA is an effective technique that trades extra receiver complexity with bandwidth and power savings. On the other hand, in systems where the number of users is high and the block size is large, storage of a high number of long interleavers may be undesirable.
SCMA addresses this complexity by using a single scrambling sequence with different seeds for different users without any performance penalty. Therefore, all of the benefits of IDMA are also true for SCMA. Similar to IDMA or random waveform CDMA [3], SCMA is a nonorthogonal multiple access technique. While orthogonal multiple access schemes such as TDMA or FDMA are implicitly too restrictive to achieve theoretical limits in fading channels, nonorthogonal CDMA, IDMA, or SCMA have the potential of achieving these limits [4]. Coding is typically used to improve the performance. At the receiver, iterative multiuser detection or interference cancellation followed by decoding is performed to approach Maximum Likelihood (ML) receiver performance without excessive complexity. But for coded CDMA systems, even this iterative receiver may lead to complicated algorithms, especially when the number of users is large. Typically with CDMA, the complexity of multiuser detection or soft interference cancellation algorithms grows in polynomial form with the number of users [5]. On the other hand, similar to IDMA, SCMA lends itself to a simple chip by a chip detection algorithm whose total complexity grows only linearly with the number of users [1]. Furthermore, uncoded IDMA or SCMA systems perform at least as well as, or usually better than, uncoded CDMA. The performance gap between the two classes of schemes grows bigger for heavily loaded systems [6].
Figure 2 depicts a general transmit block diagram and burst structure of the SCMA waveform. Each fixed-length block of information is first encoded. The code rate is significantly lower than the commonly used rates such as 1/2 or 1/3. Pilot symbols are inserted as needed periodically for receiver synchronization.
A pseudorandom number sequence is exclusive-ORed to the coded streams to provide scrambling. It also spreads the spectrum if needed. Finally, a Unique Work pattern inserted before the data is modulated into either BPSK or QPSK signals. The transmission can be in burst mode, in which case, some guard time is inserted between the bursts. It can also be transmitted in continuous mode; the guard time can then be eliminated or simply replaced with spare data as needed. Characteristics of a specific implementation have been tested successfully as summarized in Table 1.
A. Waveform in the Forward link
The 2nd Generation Digital Video Broadcast via Satellite (DVB-S2) waveform has been the standard bearer for satellite forward link applications. It employs very long LDPC code blocks (64,800 or 16,200 coded bits; 32,400 or 8,100 information bit for rate ½ coding) to achieve near Shannon-limit performance.
At sub mega bit per second, however, the latency required to transmit, receive, and decode a complete block is considerable. Furthermore, typical DVB-S2 demodulators are not designed for fast reacquisition when signal is lost, which is very undesirable for COTM applications as signals can be interrupted frequently by trees or buildings.
SCMA uses short LDPC block at a lower rate to achieve the same EbilNo threshold performance as DVB-S2 at the QPSK Rate ½ mode, although at greater bandwidth expansion, which is available anyway for MICROSAT type of applications.
Modulation | BPSK/QPSK |
---|---|
FEC code | LDPC |
Code Rate | 1/9 |
Burst length (info. byte) | 100 |
Spectrum Spreading | |
Bandwidth expansion factor (w/o spreading) | ~5 QPSK,~10 BPSK |
Single user |
1.0 |
Single User, no spreading |
−5.5 QPSK,-8.5 BPSK |
−11.5 QPSK,-14.5 BPSK |
To transmit reasonable speed of data into a small antenna suitable for COTM requires a significant portion of transponder power from a Ku-band satellite. Table 2 shows that with a typical 36 MHz Ku-band CONUS satellite transponder operating in the quasi-linear region, nearly 40 percent of the available power is required to send a 256 kbps information stream into a 7″ receive antenna, given a threshold of 1 dB EbilNo. For this data rate, SCMA and DVB-S2 would require 1.6 MHz and 320 kHz, respectively. However, since the equivalent of 14 MHz of bandwidth (BW) is required for the transponder power, the bandwidth efficiency of DVB-S2 has no practical use. In fact, concentrating 14 MHz of power into 320 kHz may violate the downlink flux density restriction. The short information block of the SCMA waveform and quick recovery from signal blockage consideration strongly favors the SCMA waveform for this application.
Antenna Size (in) | Info. Rate (kbps) | Sat. TX Power (%) | SCMA BW (MHz) | DVB-S2 BW (MHz) | Power Eq. BW (MHz) |
---|---|---|---|---|---|
7 | 256 | 38.5 | 1.6 | 0.32 | 13.86 |
10 | 256 | 17.8 | 1.6 | 0.32 | 6.4 |
12 | 256 | 12.4 | 1.6 | 0.32 | 4.45 |
12 | 512 | 24.8 | 3.2 | 0.64 | 8.9 |
12 | 1024 | 49.6 | 6.4 | 1.24 | 17.8 |
B. SCMA Waveform in the Return link
Sending
information from a very small terminal at a reasonably high information
rate requires sufficient Effective Isotropic Radiated Power (EIRP) from
the terminal. Due to lower antenna gain, more power is needed for the
same EIRP, but the beam pattern of a small antenna is too broad to meet
both on-axis and off-axis emission standards specified by regulatory
agencies. Spectrum spreading is often the only solution to mitigate the
problem by lowering the average power density both on-axis and off-axis
to achieve regulatory compliance. Use of a coding scheme with lower
The
SCMA waveform is ideally suited to address these challenges in the
return link. Since some spreading is needed to lower the spectral power
density for regulatory compliance, the bandwidth expansion may as well
be used to provide additional coding gain that can be achieved by
lower-rate codes. With the rate 1/9 code, a block of 800 information
bits can be transmitted with the same
SCMA Receiver Structures
SCMA can be used as a single access waveform in the sense that only one signal is present in an assigned frequency and time slot for either the forward or the return link. (By this definition, multiple terminals access, the same frequency at different time slot, a.k.a. TDMA, is considered single access.) The conventional receiver structure shown in Figure 3 can typically be applied.
Due
to the inherent bandwidth expansion of SCMA waveform, multiple access
to the same frequency and time slot by multiple transmissions is also
possible. The multiple signals are separated by different unique words
and scrambling/spreading sequences. The conventional receivers can still
be used for this application, one for each transmit signal. Here, each
receiver treats signals from other signals as noise. Depending on
modulation and spreading factors, the presence of other signals causes
different levels of added noise, but since they are still much lower
than the noise itself, the impact can be accepted with a moderate
increase of threshold
A more advanced receiver structure, hereafter called the SCMA receiver, takes advantage of soft-in, soft-out multiuser detection and LDPC decoding as shown in Figure 1. With this improvement, the impact on the other user on noise floor is reduced and more users can be accommodated. Another benefit of the iterative SCMA receiver structure is that the system performance actually improves with power variations among the users. This eliminates the need of power control, an important requirement with conventional receiver structure.
In the basic algorithm, FEC decoding of all
Adaptive Modulation and Spreading
Similar
to the Adaptive Code Modulation (ACM) used in DVB-S2, SCMA waveform can
easily be adapted for different channel conditions by adjusting the
modulation and spreading factor. Using the example of Table 1, we can compile a group of modulation/spreading factor that covers a range of 9 dB in the
Mod | Spreading Factor | Single Stream Es/No (dB) | No. of Parallel Streams | Multi-Stream Es/No (dB) |
---|---|---|---|---|
QPSK | xl | −5.5 | 2 | −4.2 |
BPSK | xl | −8.5 | 3 | −7.2 |
QPSK | x4 | −11.5 | 5 | −10.2 |
BPSK | x4 | −14.5 | 9 | −13.2 |
Also shown in Table 3
are the number of parallel streams of equal power signals that can
occupy the same bandwidth and time slot, with a modest (1.3 dB) increase
of
Figure 4 shows simulation results of a fixed point implementation of the conventional receiver for BPSK without additional spreading, with a single stream and with three parallel streams at equal power. The result shows the required incremental power of 1.3 dB, the same as shown in Table 3. Figure 5 illustrates the performance of equal powered QPSK streams without spreading, using the SCMA receiver. As indicated in Table 3, this mode can support up to two equal powered streams with conventional receiver. With the SCMA receiver, four parallel equal powered streams can be supported with about the same incremental power, clearly illustrating the advantage of the SCMA receiver over the conventional receiver.
Random Multiple Access with SCMA Receiver
SCMA receiver fits especially well for return links where coordination or channel assignment of transmitting remote terminals can be difficult. More importantly, an extra round trip of propagation delay is required for a terminal to request and receive channel assignment. For geosynchronous satellites, this extra step adds significant latency. For applications where each terminal has a small amount of information to transmit (such as asset tracking, credit card transactions, and smart grid), the conventional approach is to use Slotted-Aloha (S-Aloha) to bypass this step. S-Aloha, however, must be operated at very light loading, typically below 15 percent for reasonable performance and stability. SCMA, with its capability to handle multiple transmissions in the same frequency and time slot at a better success rate than S-Aloha, traffic is a very attractive alternative.
For random arrival traffic, a non-negligible probability for substantially higher simultaneous arrival than the mean arrival rate exists. This non-negligible probability dominates the SCMA receiver performance. If a less than 1 percent probability of packet error rate is desired, the SCMA receiver must be provisioned to handle a minimum of U simultaneous arrivals, such that the cumulative probability of more than U arrivals is significantly less than 1 percent. SCMA receiver complexity is a strong function of U. Not only the receiver needs to process up to U users, the number of iterations required for the processing also grows nonlinearly with U.
Table 4 summarizes the value of U for each mean arrival rate A corresponding to a cumulative probability less than 10−3.
1 | 2 | 3 | 4 | 5 | |
U | 5 | 7 | 9 | 11 | 13 |
We have optimized the design of a SCMA receiver that is capable of handling up to 13 simultaneous users. Figure 6 shows the simulated performance of such SCMA receiver with different values of arrival rate. Here, we have assumed that the received signal power is uniformly distributed around the average within [-2 dB, 2 dB].
Compared to QPSK and rate
Spread SCMA
Spreading can be applied to SCMA. However, from a performance point of view, it is beneficial to get all of the coding gain of low rate codes with the least bandwidth. To illustrate this point, Figure 7 depicts the performance of SCMA with a spreading factor of 3 and a Rate 1/5 code and unspread SCMA with Rate 1/15. The number of information bits is 160.
The advantage of SCMA with low rate coding compared to a more conventional code rate with spreading is clear. However, for applications that require high Anti-Jamming (AJ) capability, spreading the SCMA waveform can provide additional processing gain. For very small satellite terminals, spreading SCMA waveform further reduces the interferences to other satellites [8]. In either case, spread SCMA provides the required processing gain as an AJ feature between the code rate expansion and spreading with the least bandwidth occupancy. As mentioned previously, SCMA allows small terminals sending short messages at a moderate data rate with minimum latency at a very low signal-to-noise ratio. Terminals can transmit without coordination with the hub terminal or other remote terminals. They can thus be operated autonomously. This property is advantageous for applications such as Voice over IP (VoIP), which can further exploit the activity factor for statistical multiplexing gain to further optimize power and bandwidth efficiencies.
Beneficial SCMA Attributes and Applications
Unlike CDMA, SCMA allows terminals to transmit at a reasonable range of power level without coordination. In fact, reasonable burst power variation among user terminals actually improves the speed of convergence of receiver processing, and therefore, the performance. Compared to TDMA, SCMA slot timing synchronization can be less precise, as randomness of the burst arrival time actually helps the receiver to differentiate bursts transmitted by different terminals with the same scrambling code phase offset. Table 5 summarizes the benefits of the SCMA technology.
Traditionally, L-band satellites have been used for low data rate environment with small antennas. Because of the scarcity of L-band, it is more costly compared to Ku-and Ka-bands. As we are trying to broaden the applications of Ku-and Ka-band satellites to applications such as smart grid, tactical communications, mobile asset tracking, and sensor networks, it becomes apparent that these key beneficial SCMA attributes are also the desired features for these application domains. Table 6 shows the mapping between the desired features of these applications to the SCMA attributes.
Benefit | Description |
---|---|
Small Size | Power efficiency and low transmit power spectral density reduce antenna size |
Autonomy | Suited for return link without requiring central coordination and close power control |
Spectral Efficiency | More than twice the efficiency of CDMA with low rate coding and minimum spreading |
Covertness | Low spectral power density, hiding signal under noise floor |
Mobility | Short code blocks and robust synchronization for rapid recovery of signals after transmission path disruption |
Application Domains | Small Size | Antonomy | Spectral Efficiency | Covertness | Mobility |
---|---|---|---|---|---|
Smart Grid |
|
| |||
Tactical Comms | |||||
Asset Tracking |
| ||||
Sensor Network |
|
| |||
Command and Control |
A. Asset Tracking
Asset tracking is a mission-critical function for commercial enterprises and government organizations that field vehicles, ships, containers, industrial equipment, and other assets over large geographical areas.
For timely and accurate position reporting, a satellite transport accessible with a small form factor satellite terminal is mandatory. Position reporting has to be frequent to accurately map trajectories of fast-moving platforms and may require transmissions at least once per minute, which can result in over 43 million messages per month (30*24*60*1000) even for a small 1000-asset network. The resulting space segment service costs can limit the scalability of such tracking systems over spectrally limited L band (with typically <50 MHz allocation) satellite systems.
SCMA makes it possible to deploy a small form factor satellite terminal that can utilize Ku or Ka satellites in multiple access mode. With more spectral resources, Ku or Ka (with typically >500 MHz allocation) based SCMA service can often be an order or two orders of magnitude more cost-efficient compared to the L band satellite services.
B. Command and Control
SCMA can also support data rates required by text messaging, VoIP, email, file transfers, and other command and control applications. Small-size satellite terminals, based on SCMA, will be better suited for tactical units that can use a common satellite transport for their asset tracking, force tracking, and command and control needs. Besides spectral efficiency, covertness becomes another desirable feature, especially for tactical units operating behind the enemy lines. Below noise-level transmission capability of SCMA should be useful under these circumstances. Furthermore, spread SCMA can also render additional processing gain associated with wider bandwidth-enhancing resilience against jamming.
Commercial asset tracking, in conjunction with ancillary data services, has evolved into mission-critical movement tracking and (blue) force tracking functions used for defense operations. Additional data can support vehicle maintenance and diagnostics and help automate complex supply chain management and battlefield logistics. Any military organization at various hierarchical levels (e.g., platoon, company, battalion, brigade, and division) requires comprehensive and timely situational awareness of all friendly forces and various assets supporting them to assess the battlefield and make appropriate decisions.
Implementation and Field Test
A MICROSAT terminal consisting of SCMA transmitter and conventional receiver supporting a subset of the SCMA waveforms has been implemented on 1-U chassis Hughes HX260 terminals with enhanced Field Programmable Logic Array (FPGA). Each modified HX260 terminal interfaces with a 6.8″ circular-equivalent ultra small two-dimensional tracking Ku-band mobile antennas that are vehicle-mounted. These terminals have been successful in a Churchill B course, as well as., at highway speed.
Communications between the mobile terminals and a hub are maintained via a commercial Ku-band CONUS satellite throughout the test as illustrated in Figure 8. In the forward link, 512 kbps information is transmitted to the vehicles using BPSK without spreading. In the return link, 64 or 256 kbps information rate is transmitted with BPSK modulation and x4 spreading, occupying 3 or 12 MHz of bandwidth. Situational awareness and other messages were exchanged while VoIP connections were maintained between the vehicles via the hub throughout the duration of the tests. Signal interruptions, due to path blockage to the satellite or vehicle vibration caused by terrain. were recovered without noticeable lag.
An
SCMA receiver prototype was also developed for QPSK modulation without
spreading. The receiver was capable of receiving up to 13 simultaneous
transmissions in the same slot, as shown in Table 4, corresponding to a mean arrival rate of
CONCLUSIONS
We have introduced SCMA, a novel coding and modulation scheme that combines the advantages of TDMA and CDMA technologies. We described the technical details of SCMA and compared its spectral efficiency, low transmission power, and multiplexing capabilities with the existing wireless technologies, including CDMA and IDMA. These SCMA characteristics enable the use of Ku-and Ka-band satellites with very small antennas which has traditionally required operationally expensive L-band satellites.
We summarized the salient analytical and simulation results substantiating the advantages of SCMA and identified potential application areas in smart grid management, tactical defense applications, asset tracking, and sensor networking. They all benefit from the autonomous SCMA-based networks that do not require centralized power or frequency slot management and yet provide scalable multiple access operation.
We are currently enhancing our existing satellite terminal product line with the SCMA technology prototypes and have planned more enhanced demonstrations, including higher data rates and multiple land-mobile and airborne platforms, in the near future. These demonstrations will be used to validate realistic operational scenarios and provide technical data for future products based on the SCMA technology. The use of SCMA-based terminals with Ku-and Ka-band, combined with small form factor (e.g., 7″ antenna size), enables a wide range of potential applications with cost effective Ku and Ka satellites worldwide.
No comments:
Post a Comment